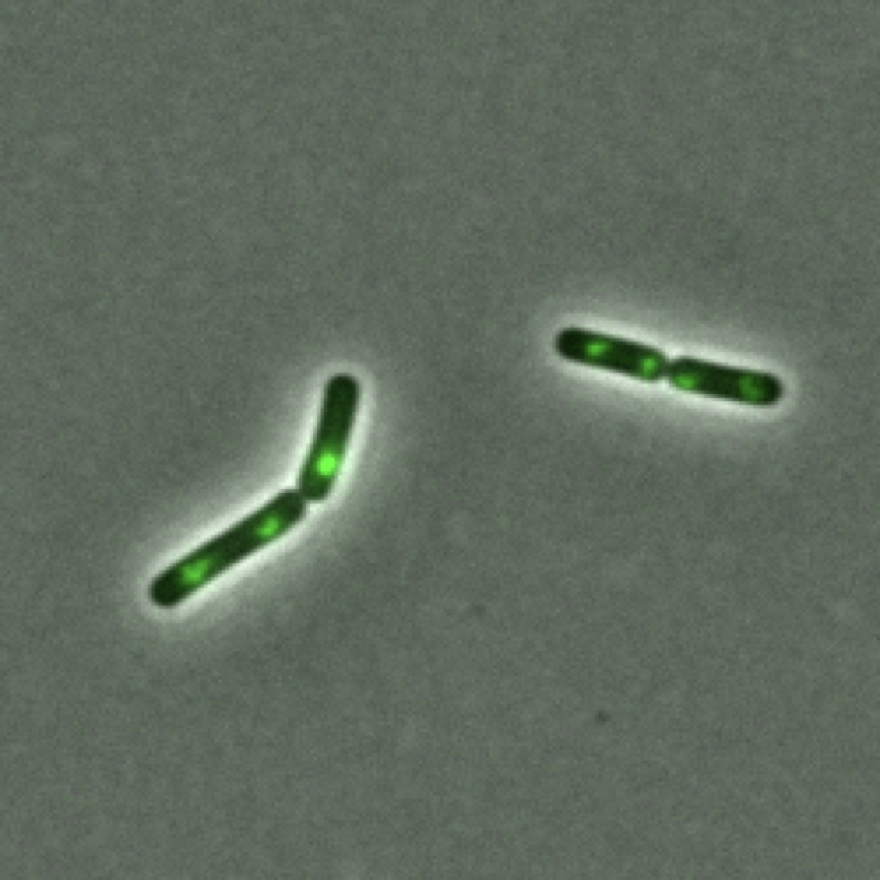
How bacteria maintain balanced growth
Cover image was collected by me and is of fluorescently tagged sites of DNA replication in B. subtilis.
Published as:
-
How bacteria link their growth rate to external nutrient conditions is unknown. To investigate how Bacillus subtilis cells alter the rate at which they expand their cell walls as they grow, we compared single-cell growth rates of cells grown under agar pads with the density of moving MreB filaments under a variety of growth conditions. MreB filament density increases proportionally with growth rate. We show that both MreB filament density and growth rate depend on the abundance of Lipid II and murAA, the first gene in the biosynthetic pathway creating the cell wall precursor Lipid II. Lipid II is sensed by the serine/threonine kinase PrkC, which phosphorylates RodZ and other proteins. We show that phosphorylated RodZ increases MreB filament density, which in turn increases cell growth rate. We also show that increasing the activity of this pathway in nutrient-poor media results in cells that elongate faster than wild-type cells, which means that B. subtilis contains spare ‘growth capacity’. We conclude that PrkC functions as a cellular rheostat, enabling fine-tuning of cell growth rates in response to Lipid II in different nutrient conditions.
Background
I joined the Garner lab as a PhD student because I was interested in understanding how individual proteins, which are only nanometers ($10^{−9}$ m) in size, are able to affect cells that are microns ($10^{−6}$ m) big. Bacteria seemed like perfect organisms to explore these questions: with their fast growth rates, it means the feedback loop of a typical experiment is days to weeks rather than months to years.
When I joined the lab one of the postdocs, Yingjie Sun, was trying to understand how the Gram positive bacteria Bacillus subtilis control how quickly they grow, and more specifically how they control the rate that they expand their cell wall. The cell wall is a rigid structure which surrounds bacterial cells and prevents the cells from lysing (a.k.a. exploding). While working on this project, he incidentally discovered that forcing cells to make cell wall faster can also cause them to grow faster in certain conditions. This was extremely surprising - the dogma at the time was that bacteria are evolved to maximize their growth rate. Because growing B. subtilis cells need to do more than just make new cell wall, I joined on the project to understand whether other essential processes (protein synthesis, DNA synthesis, division rate) were also increasing when we over-activated cell wall synthesis.
Yingjie performed a truly impressive amount of work for this project, but for the purposes of this post, I am going to focus primarily on my contributions to this work.
Introduction
Bacillus subtilis is a rod-shaped bacterium, normally found in the soil and commonly used as a model organism in the laboratory.
A phase microscopy timelapse that I took of individual B. subtilis cells doubling in size and dividing multiple times over a roughly 4 hour time course.
What I find fascinating when watching B. subtilis grow is:
How quickly they are capable of growing (A doubling time of ~20 minutes in the richest media!)
How robust the process is (all of the individuals in this movie are able to maintain the same growth rate during the entirety of the movie).
At a super high level, B. subtilis grows by taking in the nutrients from its environment and using it to make more stuff (e.g. DNA, proteins, ribosomes).
When in an environment with (effectively) unlimited nutrients, bacteria typically enter balanced or “steady state” growth, where all cellular processes proceed at the same rate that the cells are doubling in size.
In the past few decades, careful studies on quantitative bacterial physiology during balanced growth have typically been done with the bacterium E. coli [ref]. B. subtilis is similar to E. coli in a lot of ways, but one very obvious way that they differ is in their cell wall. Unlike E. coli which has a thin, single layered cell wall, B. subtilis is surrounded by a thick, multilayered peptidoglycan cell wall.
The (Gram positive) cell wall
A cross sectional view of B. subtilis. The cell wall is a the dark grey structure which separates the interior of the cell (the cytoplasm) from its environment. [source]
B. subtilis has such a thick cell wall because the cells are extremely pressurized and so without the rigid cell wall to protect it, the cells will literally explode. The cells invest a lot of resources in order to prevent lysis: the cell wall is approximately 15% of the cellular dry mass [ref]. The cell wall, however, physically limits how big a cell can be at any given time. Thus, in order to grow quickly and robustly, B. subtilis needs to carefully expand the cell wall at the same rate that everything else is growing.
I’m not going to get into the nitty-gritty of how the cell wall is synthesized, but what Yingjie found is that in certain nutrient conditions, he could increase the growth rate of cells by around 40% by over-activating cell wall synthesis (!!). Intriguingly, a point mutant in RodZ, a protein that to the best of our knowledge is only involved in cell wall synthesis, was capable of increasing cell growth rate on its own. Generally microbiologists think that bacteria try to grow as quickly as possible and that the growth rate is limited by the quality of the nutrients they are grown in. This is decidedly not the case for B. subtilis! In the conditions he tested, the rate of cell wall synthesis is limiting growth rate.
When measuring growth rate, Yingjie was specifically measuring the rate at which individual cells change their volume (single B. subtilis cells increase in volume exponentially). When growing in steady state, however, bacterial cells need to do more than just double in size: they need to double in number (a.k.a. divide into two) and double all of their cytoplasmic components. What I wanted to know was whether other essential processes (protein synthesis, DNA synthesis, division rate) was also increasing in addition to the growth rate when we over-activated cell wall synthesis.
Ultimately, I measured the rate of these essential processes using fluorescence microscopy.
Measuring protein synthesis rates by measuring the abundance of a ribosomal protein (RplL) fused to a fluorescent protein.
Measuring DNA synthesis rates by looking at an enzyme involved in DNA replication (DnaN) and measuring the number of sites of DNA replication.
Measuring division rates by looking at an enzyme that is part of the division machinery and synthesizes new cell wall (Pbp2B), separating daughter cells.
What I found is that the rates of protein synthesis, DNA synthesis, and division all increase to keep up with growth rate when we over-activate cell wall synthesis.
Protein synthesis
Proteins make up roughly half of a bacterial cell, by mass. As a result, many people interested in quantitatively understanding bacterial growth end up studying how cells decide which specific proteins to make and how many ribosomes to make. For those that are physics-inclined, the ribosome is fascinating because it is the machinery that synthesizes new proteins and the ribosome itself is made up of proteins. Because ribosomal production is autocatalytic, people coarse-grain a bacterial cell as being a bag of full of ribosomes dedicated to synthesizing more ribosomes. This also leads to a very natural explanation of why the growth of single B. subtilis cells is exponential: if the rate of ribosomal production depends on its own abundance, ribosomes will increase exponentially.
In order to see if protein synthesis rates were increasing in these fast growing mutants, I first approached this question indirectly, by measuring the rate of mass increase. Since most of the cell mass is protein, I reasoned that if cells are making cell mass more quickly, they are likely also making proteins more quickly. To make these measurements, I turned to quantitative phase microscopy (quick primer on quantitative phase microscopy).
I measured both cell mass and cell volume simultaneously over time, and then calculated the rate that individual cells are doubling in size and doubling in mass.
Rate of cell volume increase (x-axis) and rate of cell mass increase (y-axis) for wild-type and PrkC over-expression strain. Each point represents a measurement from an individual cell.
When I make this measurement, what I see is that over-expressing PrkC leads to cells that, when compared to the wild-type strain, both increase in cell mass and cell volume faster. This tells us that the fast growing strains are probably also making protein more quickly.
Because protein synthesis is catalyzed by ribosomes, the field of growth rate regulation has taken on a very ribosome-centric perspective. It has been known for decades there is a linear dependence between ribosomal abundance and growth rate for many bacteria, including B. subtilis. In other words, the faster a cell is growing, the more ribosomes it needs to support that growth rate.
Model of growth rate v. ribosomal abundance scaling if cells were utilizing spare capacity. Blue is WT data in different media, and orange is mutants data.
Division and growth rate are the same and so cell size homeostasis is maintained.
Correlation between growth rate and RNA/protein ratio in E. coli growth in different media. RNA/protein ratio is often used as a proxy for ribosomal abundance. [source]
Notably, the y-intercept of this curve is not zero, which has been interpreted to mean that not all ribosomes are being utilized. This spare capacity is useful because in changing environments, bacteria can deploy some of the “spare” ribosomes to quickly change protein expression.
I figured that there were two ways that B. subtilis could increase protein synthesis when we over-activated cell wall synthesis: a) utilizing spare ribosomes, making the cells less adaptable in the process, or b) making more ribosomes. To be honest, we were expecting that these cells would be utilizing spare ribosomes since we figured that this faster cell growth had to come at the expense of something.
Model of growth rate v. ribosomal abundance scaling if cells were making more ribosomes. Blue is WT data in different media, and orange is mutants data.
Division rate is faster than growth rate and so the cell decreases in size over time
Actual ribosomal abundance vs. growth rate data for wild type in different media (blue) and over-activated cell wall synthesis strains (orange).
What we saw though was that when we over-activated cell wall synthesis, the cells make more ribosomes than their wild-type cells in the same media. This tells us that protein synthesis rate and ribosomal abundance increase in addition to growth rate when we over-activate cell wall synthesis.
DNA synthesis
When cells grow and divide, they also need to duplicate their genetic material, DNA. The B. subtilis chromosome is circular and typically DNA replication starts at a single locus on the chromosome, the origin of replication (referred to also as simply “the origin” or “ori”).
A bacterial cell growing, dividing, and replicating its chromosome. The chromosome is depicted as the black circle and the origin of replication is depicted as the red dot. When the cell is replicating its chromosome, there are two active sites of DNA replication.
n B. subtilis, people have found that if you measure the number of origins that a cell has and divide it by the total size of a cell, the number of origins per cell size is constant. I ended up making a slightly different measurement: instead of counting the number of origins, I imaged the fluorescently tagged a component of the DNA replication machinery and counted the number of active sites of DNA replication in each cell. At the growth rates that I am studying, however, I that ~all of the chromosomes to be undergoing replication at any given time, and so this is a reasonable proxy for the number of origins in a cell.
While measuring the cell size is relatively straightforward, measuring the number of active sites of DNA replication that a cell has using microscopy brings some technical challenges. Specifically, because of the diffraction limit I am only able to distinguish objects if they are at least 250 nanometers apart. Considering that the average protein is only ~10 nanometers in size and B. subtilis is only 750 nanometers wide, this means that even if there are two sites of DNA replication that are relatively far apart they still might be difficult to distinguish.
Luckily, Suckjoon Jun’s lab has published a method for doing precisely this. Briefly, they identified fluorescent foci and then quantified the total fluorescent signal from that foci. They then fit a Gaussian mixture model to the distribution of fluorescence intensity of all foci. Typically the position of the second peak generally corresponded to two times that of the first peak, suggesting that the second peak corresponds to two fluorescence foci that are spatially overlapping and undistinguishable due to the diffraction limit. Using the Gaussian mixture model, they classified spots as either corresponding to one or two foci depending on whether or not it had a higher probability of falling into the first or second peak region.
By adapting their methods, I was able to count the number of sites of DNA replication
The number of sites of DNA replication (as measured by looking at DnaN foci) per volume for wild type and over-activate cell wall synthesis strains.
What we see is that the number of sites of DNA replication per volume remains constant, telling us that DNA synthesis rate is increasing in addition to growth rate when we over-activate cell wall synthesis.
Division rate
In the words of François Jacob, “the dream of every cell is to become two cells”. During a cell cycle, a cell both doubles in volume (going from ~1 femtoliter to ~2 femtoliters) and doubles in number (going from 1 cell to 2 cells). The rate that a cell doubles in size is given by its growth rate and the rate that a cell doubles in number is given by its division rate. Notably, single cell growth of B. subtilis is exponential, and as a result for a given growth rate the amount of time that it takes for a cell to double in size is independent of how big the cell is at birth.
For a cell to be in balanced growth, the growth and division rate need to be the same. If the growth and division rate are not the same, then cell size homeostasis will not be maintained. This becomes readily apparent if we model the size of a single (noiseless) cell as it grows exponentially and divides and vary the relative rates of growth and division.
Division rate is slower than growth rate and so the cell increases in size over time
What we see when we measure the growth and division rate of wild type cells and cells that have over-activated cell wall synthesis is that in all cases, division rate keeps up with growth rate.
Thus, division rate is increasing in addition to growth rate when we over-activate cell wall synthesis.